Welcome to Climate Drift - the place where we explain climate solutions and help you find your role in the race to net zero.
If you haven’t subscribed, join here:
Hi there! 👋
Skander here.
Our Climate Drift Career Accelerator for mid-career & executives is now in its 8th week and this week we are sharing some deep dives focused on microgrids, starting with a primer by Peter.
Peter Blair is a social impact leader with 12+ years building teams and operations at nonprofits, social enterprises, and Salesforce.org. He’s diving with us into a primer on Microgrids: What they are, the different types, examples, standards and the future.
If you want to make a difference and bring your talent into climate: Apply to our next cohort and join the Climate Drift community.
Let’s dive in. 🌊
The grid is not ready for the explosive growth in EV sales we expect to see over the next decade. While Virtual Power Plants (VPPs) will play an important role in managing the increased demand for power, we will need physical investment to make the grid more resilient. Microgrids offer the potential to fast-track a grid that can handle the increased demand for clean electricity. Read on to find out how microgrids can accelerate the resilient grid of the future, learn whether microgrids are as clean as you think, understand the regulatory challenges of a decentralized grid, and learn what challenges we still need to solve.
In this article we will cover:
Key microgrid terminology
What is a microgrid and how big are they?
Gray vs green microgrids
Examples of microgrids accelerating grid resilience
Microgrid standards and the regulatory environment
What’s next for microgrids?
As a reminder, before we jump into microgrids, the US grid is really a series of independent system operators (ISOs) as shown below. This creates an inherent challenge in that currently there is no ability for certain areas of the country to receive power from other ISOs in the event of a major service disruption. It also means that there is no way to share and redistribute load across different areas of the country to account for increased demand or unexpected shortfalls in power generation.
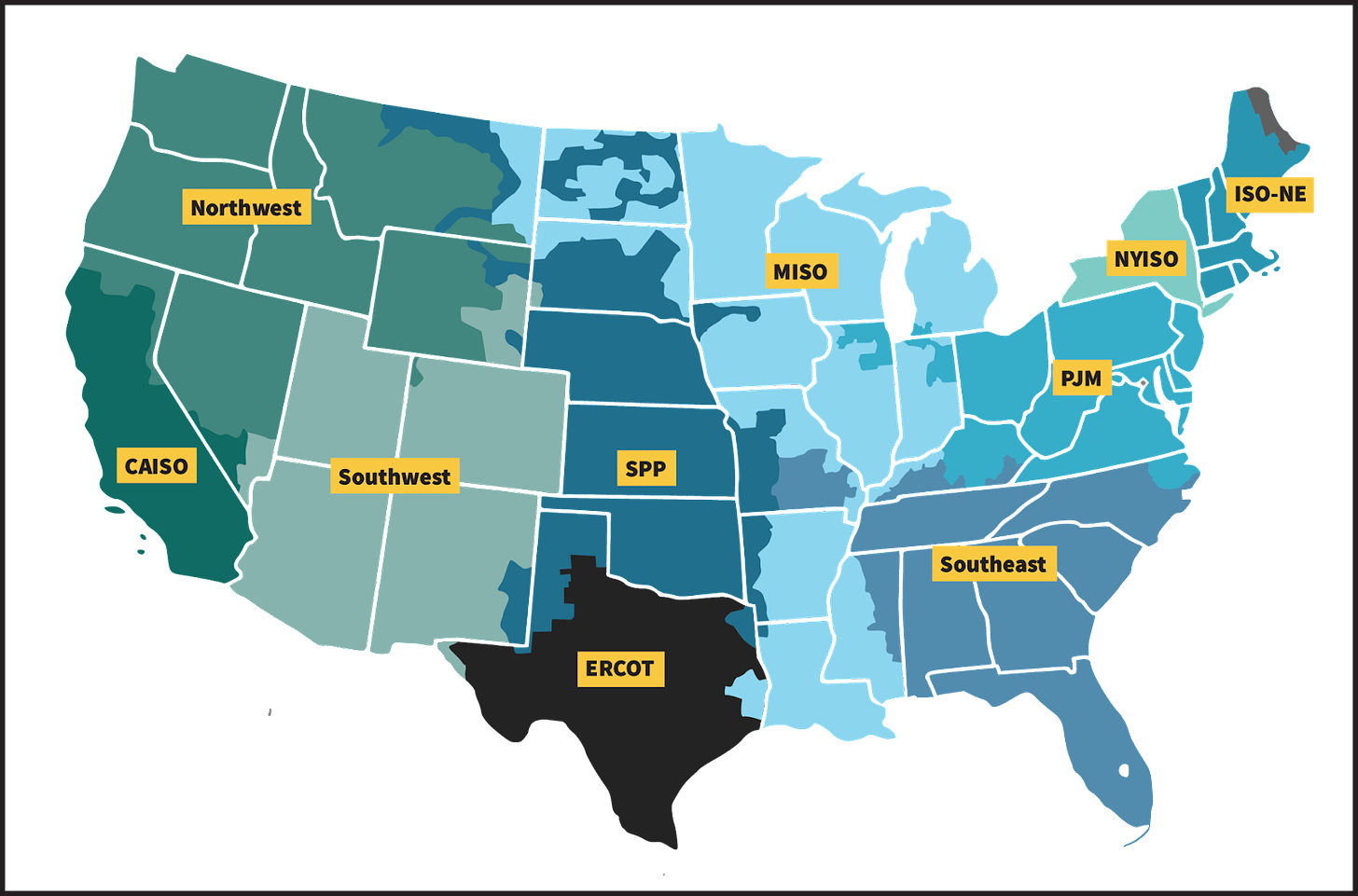
Key terminology:
Behind-the-meter - energy systems located on the customer side of the utility meter (can include solar panels, battery storage, or smart chargers).
Decarbonization - power generation that is free of fossil fuels.
Distributed Energy Resources (DERs) - any hardware device that produces or consumes electricity (more info here)
Front-of-the-meter - systems on the utility side of the meter (including large-scale energy generation, grid infrastructure, transmission, and distribution.
Reliability - the capacity to avoid power disruptions.
Resilience - the ability to withstand and quickly recover from power outages.
Switchgear - power-conducting components and control systems that allow energy to be supplied from the grid or from the microgrid in the event that the microgrid is islanding.
Virtual Power Plants (VPPs) - A pool of actively controlled DERs used to shift or reduce demands on the grid (A guide to VPPs).
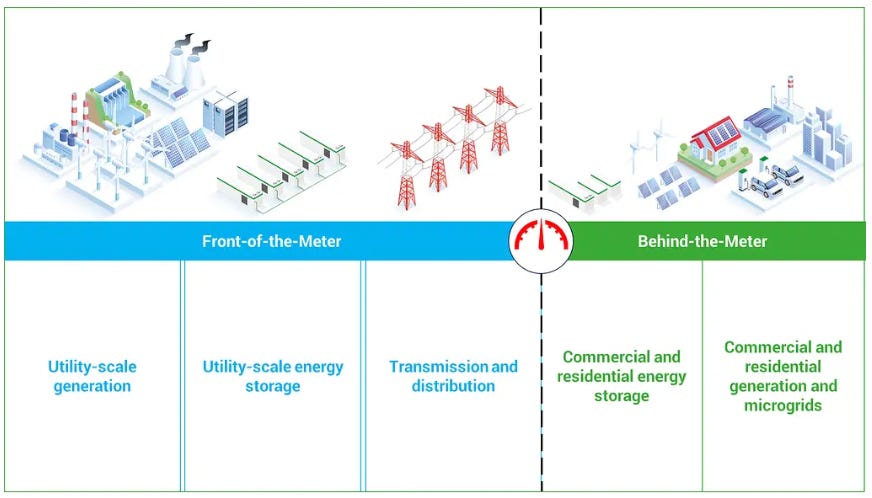
Microgrids have the potential to impact energy flow both behind-the-meter - by incorporating energy generated by distributed energy resources owned by customers and business - and supporting grid-level load demands occurring front-of-the-meter. As demands on the grid continue to rise in an electrifying society, grids will need to modernize to withstand increased need for electricity.
What is a microgrid?
Microgrids generate power and store it locally, and in the event of grid disruption can isolate from the grid to distribute power. Microgrids have the potential to play a key role in creating a more resilient grid infrastructure that is decarbonized, able to withstand and recover from outages created by weather events, and prevent service disruption. By generating power at a more localized level, they can also be more responsive to customer demand, and offset some of the losses that occur due to power transmission over long distances (around 5% of power is lost during transmission on the lines). In 2023, there were 692 microgrids in the US, generating 4.4 giga-watts of electricity (a small fraction of the US total energy generation capacity of 1,250 giga-watts).
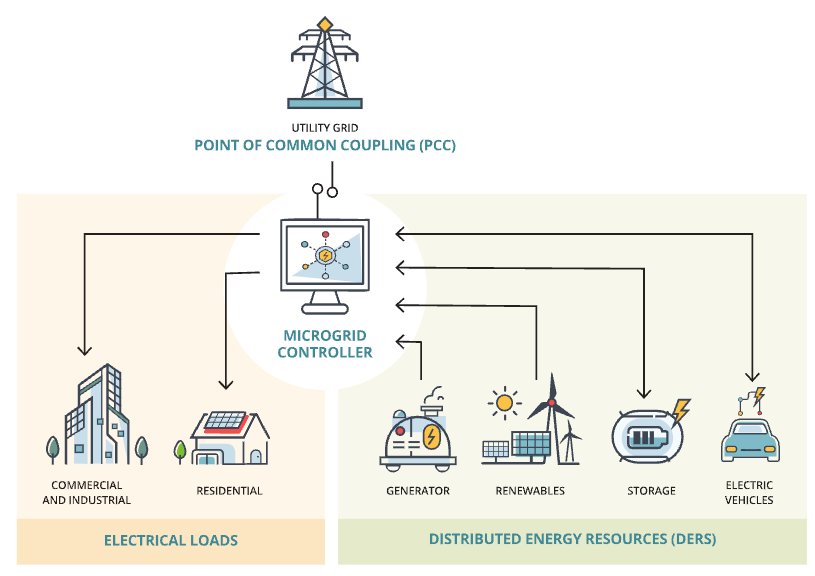
A microgrid can:
Generate power (through solar, wind, natural gas etc.)
Store power*
Isolate or “island” itself from the grid
Distribute energy stored locally
*Some facility level/small-scale microgrids do not store power and immediately use power as it is generated (for example a hospital using diesel generators during an interrupted power supply from the grid)
As opposed to backup generators, microgrids are designed to run continuously - offsetting energy demands using local resources, and storing renewable energy when it is plentiful. If power is lost from the grid, the microgrid can then operate in island-mode - providing independent power locally.
A key aspect of microgrids is their ability to prevent damage to critical infrastructure for goods and services. This can be in ensuring cold-chain storage is uninterrupted in the case of warehouses or vaccine inventory, or critical life support systems in hospitals.
Different sizes of microgrid
The broad range of use cases for microgrids mean that they can vary considerably in size - and from solving for single problems (grid disruption due to planned downtime) to a host of energy demand situations. The unifying element in all of these is the ability to generate and store power while the grid is operational, and then island and provide power if the grid is under strain or non-operational.
In general, microgrids can be thought about at three levels:
Single facility (hospital, data center, prison)
Single microgrid (serving a discrete neighborhood)
Network microgrid (a series of connected microgrids comprising generation and load management across multiple locales)
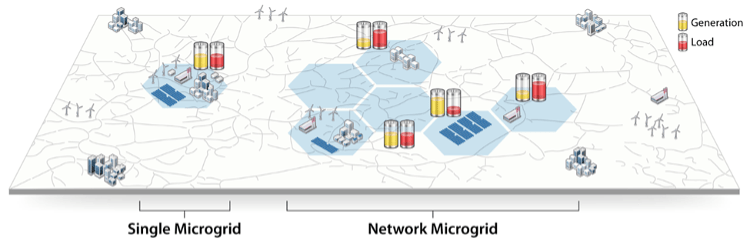
Additionally, some public utilities (such as New Jersey) have established definitions based on number of owners, facilities, and points of interconnection to the grid. One challenge that the current atomized approach to microgrid construction poses is that not all single facility- or single microgrid-level microgrids could be incorporated into broader networked systems.
Gray vs green microgrids
Much of the focus on microgrid technology has been on the decentralized and independent nature of their power supply. However, it is important to note that often little distinction is made between green microgrids and gray microgrids. In Texas for example, currently the “vast majority of the roughly 1,300 megawatts of ‘registered” DER participants.. are natural-gas- and diesel-fired generators”.
Gray microgrids can be considered any microgrid where power generation is the product of the combustion of fossil fuels. While they help solve the problems of reliability and resilience, they do not contribute to decarbonization.
For commercial microgrid applications, often microgrid as a service companies (such as Scale Microgrids) will include dispatchable power generation. Typically these assets are fossil fuel generators (either diesel or natural gas). Enchanted Rock utilize “renewable natural gas” (RNG) - treated methane from landfills, farms, or waste treatment plants - in place of natural gas for their dispatchable generators. However, RNG is still a fossil fuel and its combustion releases carbon-dioxide, with any leaks in the RNG supply chain emitting methane into the atmosphere. More details on RNG can be found in the World Resource Institute’s white paper.
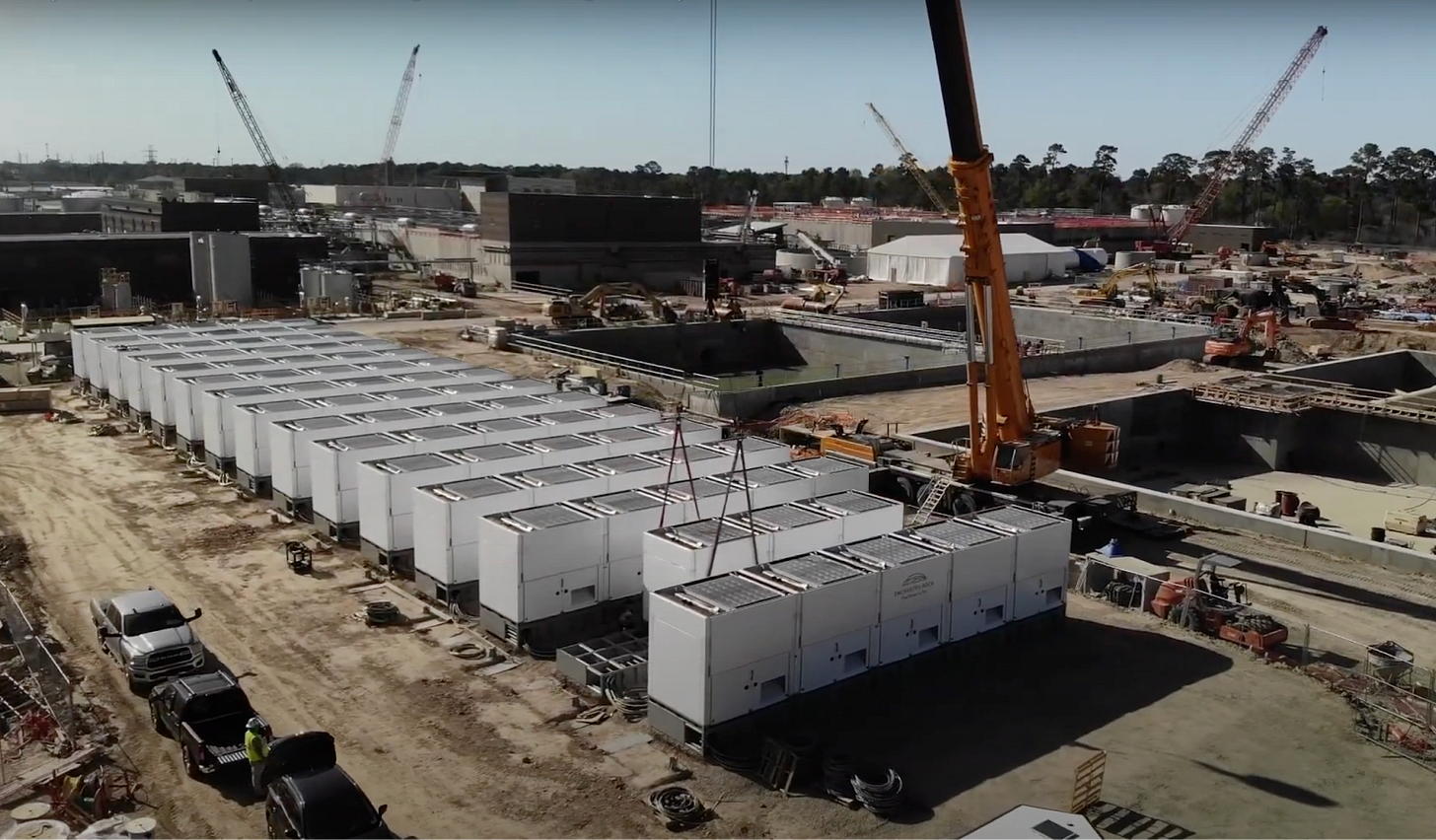
Green microgrids generate their energy solely through renewable energy sources. A key aspect of successful microgrid deployment is their need for 24/7 availability, which can be more challenging when using renewable energy sources. However, as we continue to see improvements in energy storage (falling prices, alternative battery technologies, safer hydrogen storage) we have the technology already to move away from fossil fuel powered microgrid infrastructure. As companies like Ampd Energy provide green alternatives to fossil fuel generators, we may also see a rise in dispatchable generation that is renewable energy based.
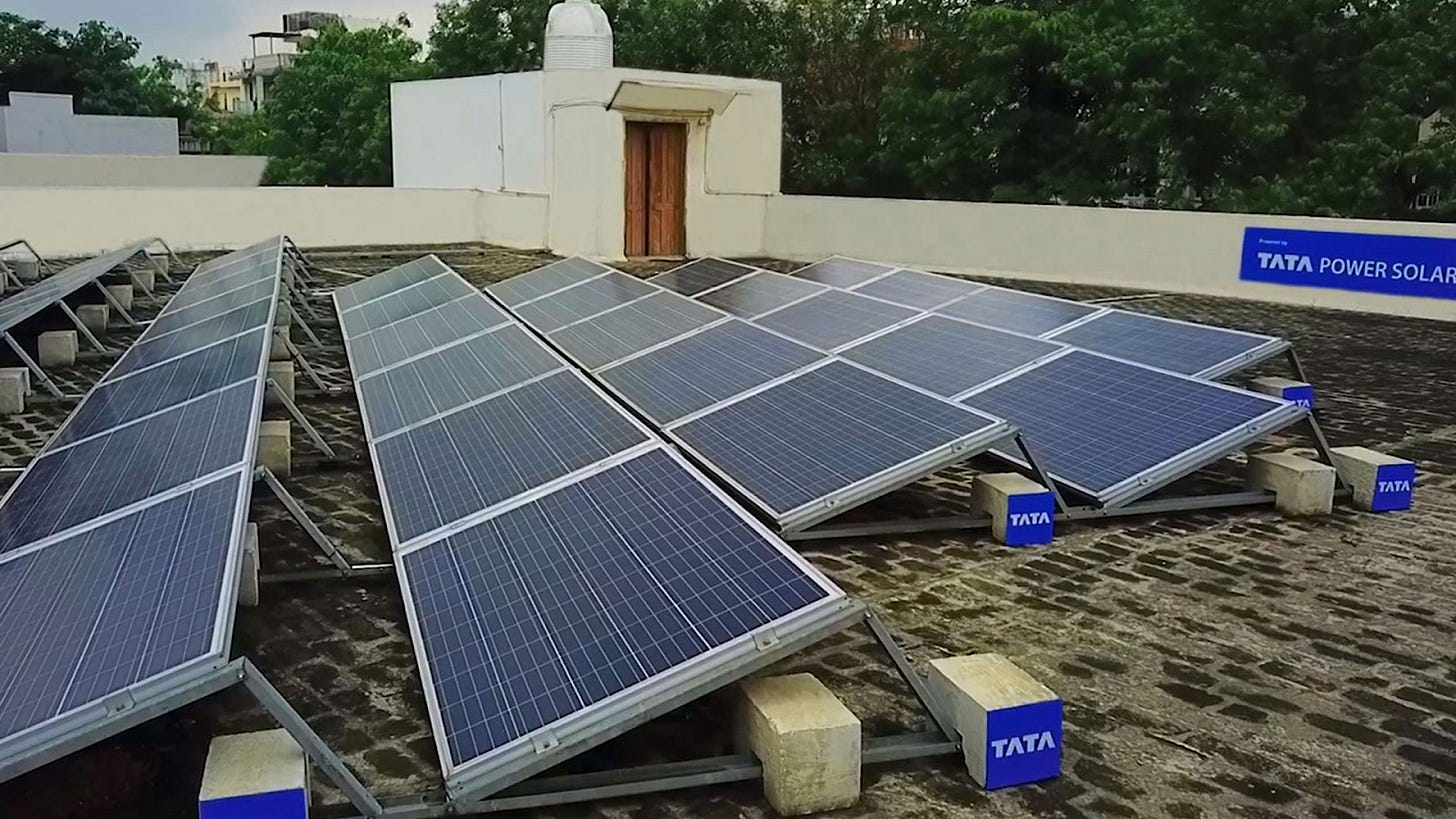
Given that energy storage is finite in microgrid environments, it is unlikely that we will see widespread blue microgrids which are powered by natural gas for generation and include a carbon capture and storage (CCS) element. Research is being devoted to how low-carbon microgrids can incorporate CCS at the facility level - such as in ports - by using carbon capture to treat the carbon emissions from the fossil fuel generated power plant while in grid-connected mode. These hybrid models where power is stored from grid generation or when renewable energy is plentiful have the potential to contribute to decarbonizing grids and providing additional reliability and resilience.
Microgrid examples - From the Pacific and big-box stores to JFK Airport
American Samoa’s diesel free microgrids
In American Samoa, Ta’u Island’s microgrid has completely replaced the island’s use of diesel generators with a solar battery storage system. The Hawai’i-based Mana Pacific designed and implemented an installation in partnership with SolarCity and Tesla comprising 5,300 solar panels, capable of generating 1.4 megawatts of power, with 6 megawatt-hours of battery storage, powering the island for three days without sun. The solar panels were also designed to withstand Category 5 hurricane force winds. Mana Pacific is working to construct additional capacity across the Pacific islands on Hawai’i and Fiji.
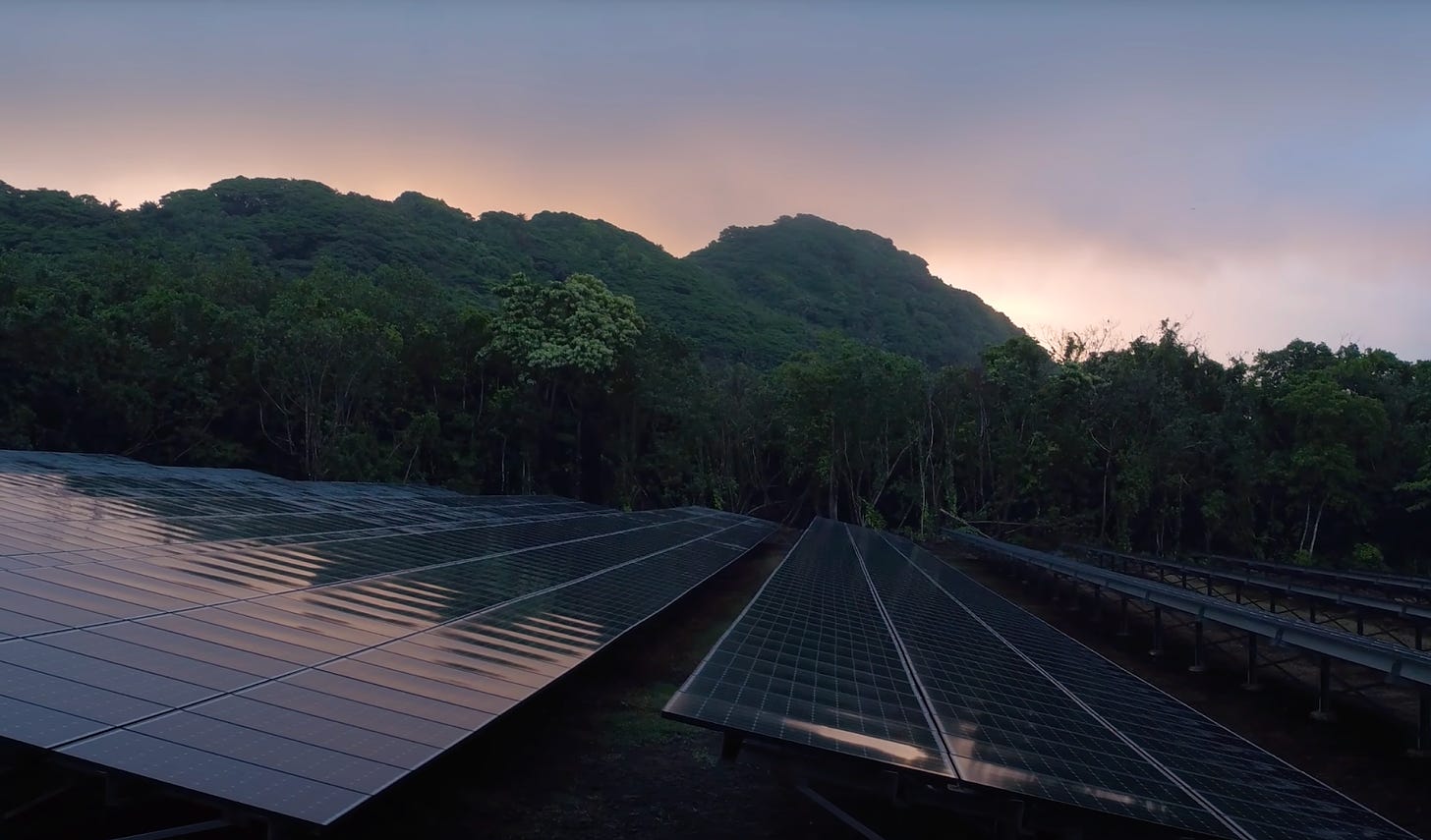
Walmart’s path to 100% renewable energy
As part of its Project Gigaton commitment (Walmart’s effort to avoid one billion metric tons, or one gigaton, of CO2 emissions from global value chains by 2030) it is committed to moving to 100% renewable energy by 2035. Walmart committed to investing in microgrid technology in 2017, entering into an agreement with Advanced Microgrid Solutions (acquired by Fluence/Schneider Electric in 2020) to provide 40 megawatt-hours of behind the meter battery storage. The creation of hybrid electric buildings creates the ability for local utility companies to tap into their stored power and reduce load on the grid. This also allows Walmart’s to sustain operations at its own facilities in the event of grid-level power disruption. In addition, Walmart created the Gigaton purchasing power agreement (GGPA) which educates companies interested in investing in renewable energy and make purchases as part of a larger cohort. The first cohort of suppliers in the GGPA is purchasing wind power from Ørsted’s Kansas-based Sunflower Wind project.
These investments in building out not only facility level resilience for their locations, but also in building a renewable energy ecosystem that can both support the grid and decarbonize their supply chain highlights the role that large corporations and microgrids play in creating a more resilient clean energy future.
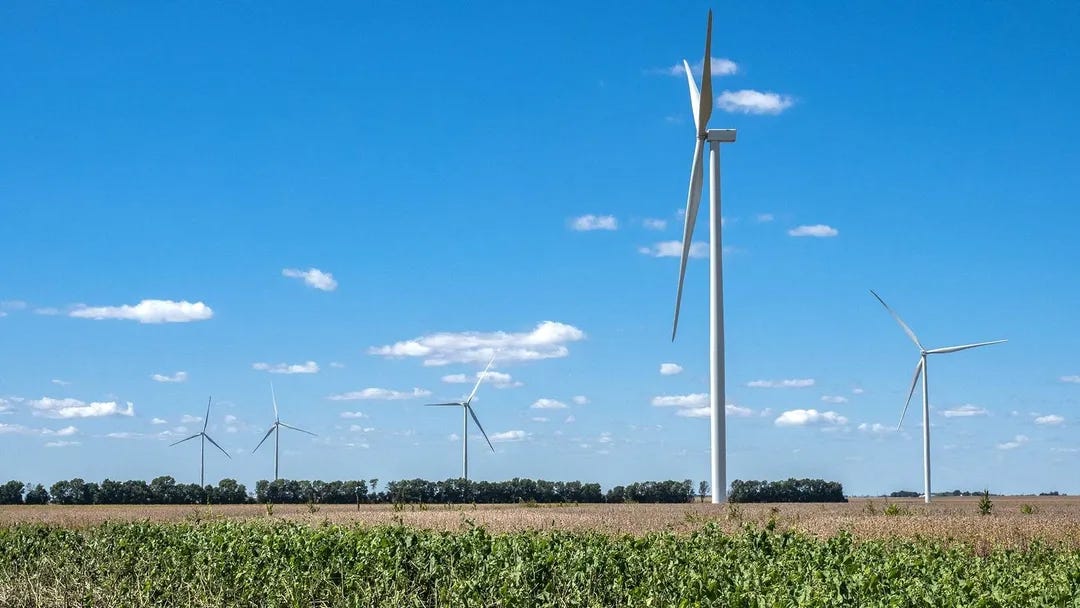
JFK Airport’s blackout-proof terminal
As part of its construction of New Terminal One (NTO), Schneider Electric and AlphaStruxure is building an 11.3 megawatt microgrid that will enable the terminal to continue to operate in the event of a blackout. The development will include 7.66 megawatts of rooftop solar, 3.68 megawatt fuel cells, and two 4 megawatt-hour batteries for energy storage. It will be the largest solar array in New York City and the largest of any US airport terminal.
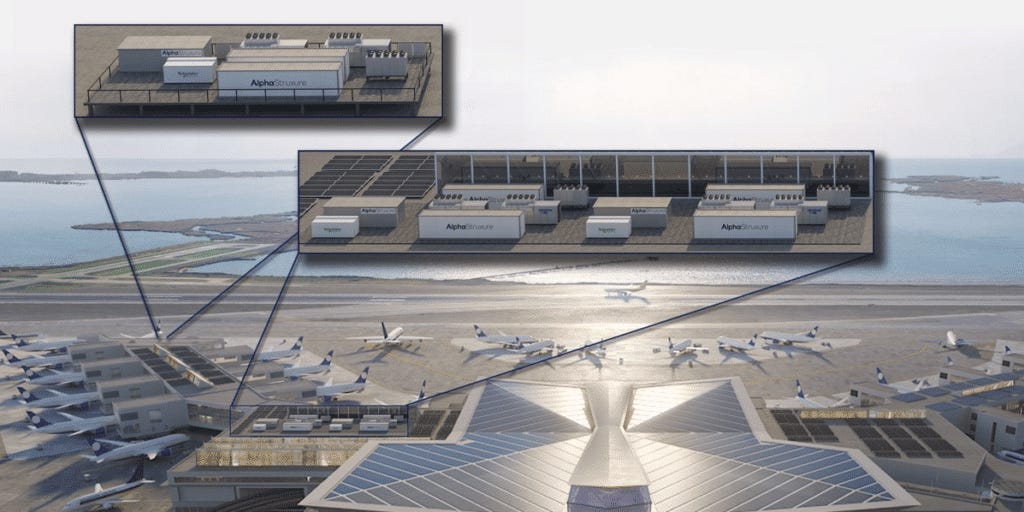
It is important to note that JFK plans to use natural gas fuel cells, similar to Pittsburgh Airport’s gray microgrid (which combines solar and natural gas production), but hopes to transition to biogas or hydrogen in the future. One key advantage of microgrids over traditional diesel generators (other than decarbonization) is that they prevent the initial power loss and disruption to services typically seen when airports experience a blackout.
The microgrid regulatory and standards environment
Currently there is a lack of national legislation and regulation for microgrids. This presents challenges to developers, funders, and most importantly to utility providers themselves. Only California, Hawai’i, Maine, and Puerto Rico have substantive microgrid laws.
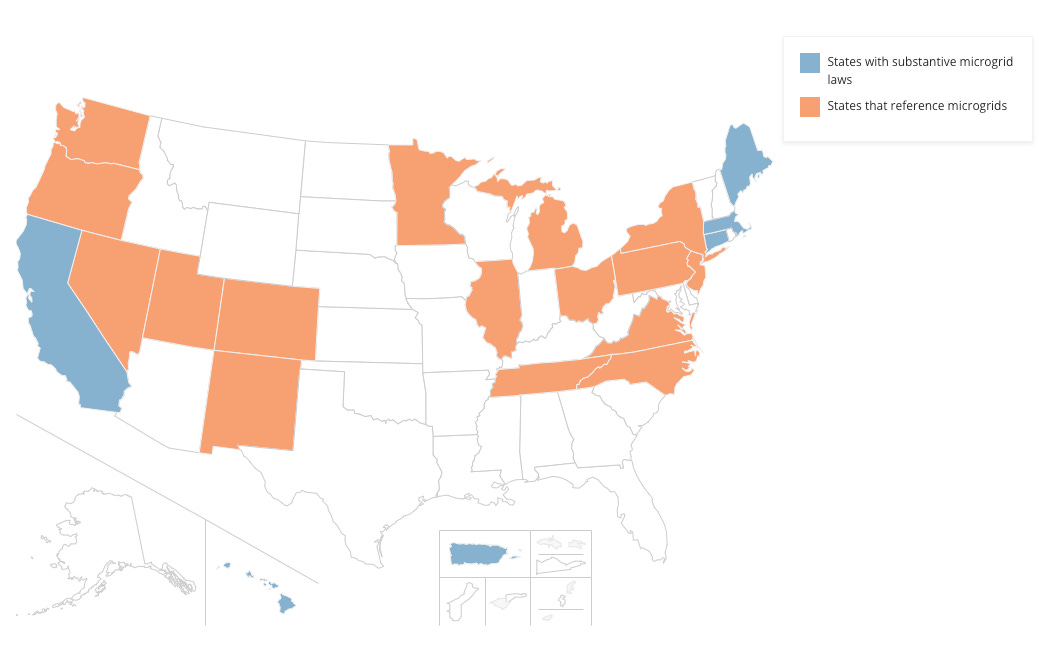
The lack of federal standards around microgrids mean that companies seeking to provide microgrid services are bound by different standards and legislation depending on which states they are operating in. Both Hawai’i and California have enacted legislation to establish microgrid tariffs, and standardize permitting and interconnection. Beyond the US, there is no global regulatory standard that applies to microgrids, as each microgrid component (such as solar PV panels and inverters). This means that component standards can act in conflict, rather than in concert.
Core standards that will be required to overcome these challenges have been proposed by researchers from the University of Madrid:
It is hard to envision a system that allows for a greater degree of interoperability between privately-, publicly-, and utility-owned DERs without a more clearly articulated standard for interconnection. The current regulatory environment comes from the deeply entrenched history of electricity management as a unidirectional consumption relationship between utility providers and consumers. The complex and decentralized future of energy production and consumption means that regulation will be required to protect aging grid infrastructure, while also allowing for innovation and resilience at a local level.
What’s next for microgrids?
Microgrids will undoubtedly play a key role in creating Grid 2.0. However, at present their deployment ranges from transformational and strategic, to haphazard and a continuation of existing fossil fuel reliance.
The IRA offers huge incentives for the development of a broad range of renewable energy generation technologies. However, there is a real risk that letting a thousand flowers bloom will lead to a fractured and messy energy sector five years from now. Microgrid construction offers a very clear opportunity for collaboration between energy generation, storage, charging infrastructure, and utility providers at the state level. Lessons can be taken from legislation in California and Hawai’i and directly applied in other contexts to accelerate the path to a more resilient grid.
Unlike the race for autonomous vehicles which is likely to see one or two winners, there is space for many winners amongst companies building technologies that can create microgrid infrastructure. As a result, creating unified positions on key issues such as interconnection and tariff standards together will allow DER providers to better work with local authorities and utility providers to create opportunities for networked microgrids that help support and decarbonize the grid.
Questions for the future of microgrids:
What would incentivize utility providers to invest in solving the problem of incorporating behind-the-meter energy generation and storage at the local level?
What technology innovation is needed to better incorporate DERs behind-the-meter?
How can more modular assets (batteries and switchgear) allow for rapidly deployable microgrid technology in the event of large scale unplanned outages?
Join the Climate Drift Accelerator and turn your passion into action. We are selecting people for our next cohort now, and we're looking for talented individuals like you to make a real difference.
🚀 Apply today: Be part of the solution
Great article, thanks for sharing. Agree completely. In terms of your qs, what technologies are needed to see more deployments…. Most energy consumers do not know they have options beyond grid only power. Beyond that, they do not know what is possible, where to begin, what facilities are good candidates or who to work with. All the hardware technologies, financial mechanisms and incentives exist today to ensure microgrids are super efficient and economical for managing businesses and communities. I believe we need more enabling technologies to inspire businesses to invest and act. This is our mission at www.veckta.com - where to build, why you should build, what you should build and then who you should build it with - automated and enabled with software.
As more systems are deployed the benefits will be realized and celebrated, systems will come down further in price and more will adopt. Shifting from passive consumers to active users - in a commoditized busienss environment this is an incredible way to realize more profitable outcomes. Utilities (in their form today but likely having needed to change their commercial models) will need to then embrace, incentivize and figure out how to best manage these incredibly powerful, flexible and dispatchable assets. Exciting times. $68B/yr market, ready to take off!